Welcome, Guest |
You have to register before you can post on our site.
|
Online Users |
There are currently 61 online users. » 0 Member(s) | 59 Guest(s) Bing, Google
|
Latest Threads |
Two Moonset times in the ...
Forum: General Topics Here
Last Post: rascalsailor
06-25-2024, 12:48 PM
» Replies: 4
» Views: 678
|
Old no-plotting method ...
Forum: The Sight Reduction process
Last Post: Rumata
03-12-2024, 04:45 AM
» Replies: 7
» Views: 6,372
|
Hello
Forum: The Sight Reduction process
Last Post: PeterB
11-23-2023, 09:39 PM
» Replies: 11
» Views: 5,867
|
Electronic Charts: New Bo...
Forum: Equipment- Wanted or for Sale
Last Post: PeterB
10-15-2023, 01:37 PM
» Replies: 0
» Views: 671
|
assumed longitude
Forum: General Topics Here
Last Post: Rumata
10-07-2023, 10:34 PM
» Replies: 2
» Views: 1,699
|
What Is Your Favorite Wat...
Forum: Equipment- Wanted or for Sale
Last Post: craigsl
09-02-2023, 10:28 PM
» Replies: 3
» Views: 2,535
|
Camp Runamuck- TV show
Forum: Humor & Laughter
Last Post: craigsl
09-01-2023, 11:20 PM
» Replies: 0
» Views: 1,010
|
Help With an Exam Questio...
Forum: Humor & Laughter
Last Post: CarlosPindle
05-08-2023, 11:30 PM
» Replies: 1
» Views: 1,521
|
USCG Crazy Exam question:...
Forum: General Topics Here
Last Post: Fred_B
05-05-2023, 09:48 PM
» Replies: 3
» Views: 2,665
|
How to get LHA and Ap lon...
Forum: General Topics Here
Last Post: Fred_B
04-29-2023, 11:16 AM
» Replies: 15
» Views: 8,296
|
|
|
Why Great Circles Are Great |
Posted by: PeterB - 04-14-2022, 09:03 PM - Forum: General Topics Here
- No Replies
|
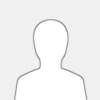 |
I have many books on celestial navigation and none of them explain the simple elegance of great circles as used in c-nav. That is a shame because they are easy to understand without any fancy mathematics whatsoever. I personally didn't “get” celestial until I figured out for myself how these great circles work. That is why I am posting this with the hope that some of you will find the following helpful – or at least interesting.
Many of us started out in celestial with the analogy of a flag pole and how the top of the flag pole appears at lower angles the further we stand from its base. This is a flat-Earth analogy that can only take you so far and sooner or later runs into limitations.
To begin with we must understand that all of the stars are so far away that the direction in which we see any stars doesn't change with our location on Earth.
For the moment consider Polaris, which seems to stand nearly still as the other stars rotate around it. If the entire Earth were the size of the period at the end of this sentence at that scale Polaris would be many times further away from the ink dot Earth than the Eiffel Tower is from New York City's Central Park. Clearly taking the bearing from one side of the ink dot in Central Park to the Eiffel Tower and then moving all the way to the complete opposite side of the ink dot and taking it again would not change the bearing any measurable amount.
In fact the real distance to Polaris is so far that even if you wait six months for the Earth to reach the opposite side of its entire orbit around the sun, the direction to Polaris won't change. What causes the change of the angle at which you see Polaris is a change in what you perceive as “horizontal” or “straight up” as you move about on the spherical Earth.
If we could transport ourselves far out into space and look back at Earth to see three people taking simultaneous observations of Polaris we would see that they are all looking in the exact same direction – at a star that appears to us to be directly over the Earth's north pole but which is incredibly far away. From our outer space location we would see that all three would be gazing northward in a direction parallel to Earth's polar axis. But Paul at the north pole sees the star directly over his head; Eddie at the equator sees it low on his horizon; and Matt in the mid latitudes sees it part way up his sky. What is different for each of them is what they consider to be “straight up” and “horizontal” depending on where they are standing on the sphere that is Earth.
This is true for all the other stars as well. It is only the spinning of the Earth that gives them the appearance of motion. If, from our outer space location, we took a look at several people taking simultaneous observations of Arcturus we would see that they too would all be looking in the exact same direction at that star, but the heights at which they each perceive the star in their local sky would differ by their locations on the sphere that is Earth.
Arcturus travels over the Big Island of Hawaii each day, so let us say we watch a bunch of people taking sights of Arcturus just as it is directly above Hawaii. To get a good mental picture of this imagine that you have a globe in your hands and hold it so that Hawaii is straight up. Now from your perspective all of the observers will be looking “straight up” at your ceiling and far beyond to see Arcturus regardless of where they are standing on the globe. Of course the further they are on the globe from Hawaii the more their personal sense of horizontal will be tipped over. If a few of them observe Arcturus at the same angle up in their sky we could say that they were all standing on a circle of position determined by the same distance from the star's geographic position. Alternately we could say that they are all standing on the same circle of position determined by an equal amount of horizontal tilting from the 0° tilt that occurs at the geographic position of the star.
Now on to great circles.
A great circle is a specific concept in spherical geometry. It is the circle that is formed on the surface of the sphere by a flat plane that passes through the exact center of the sphere. All great circles on any particular sphere have their centers at the center of the sphere. They all have the same diameter as the sphere, and they all have the same circumference, which is the maximum circumference of the sphere.
Think of cutting an orange exactly in half with a sharp straight knife. The outer edge of the peel on each of the two halves is now a circle. If the cut went through the exact center of the orange this circle would be a great circle on the orange. If instead that sphere were a beach ball it is a great circle on the beach ball; If the sphere were a classroom globe that circle would be a great circle on that globe; If the sphere were the Earth it is a great circle on Earth.
Any plane that does not go through the exact center of the sphere still makes a circle where it intersects the surface, but that is not a great circle. Instead it is technically a “small circle” even if it is enormous in size. All lines of latitude save the equator are small circles on Earth. The flat planes that any of them lie upon cut across the Earth's polar axis at some distance north or south of the actual center. Nearly all circles of position that you plot as Sumner lines or LOP's in celestial are also small circles. Small circles do not share the handy characteristics of great circles, so be sure not to conflate the two.
For our purposes it is useful to alter our perspective a bit. We say that any circle on a sphere that has the same circumference as the maximum circumference of the sphere will lie in a flat plane that cuts the sphere exactly into two halves passing through the center of the sphere, and is therefore a great circle.
Imagine a schoolroom globe of the Earth. Now imagine you have made a bracelet that slips down over this globe so that it sits exactly on the equator. The plane it lies in is the equatorial plane of the globe which we know cuts the globe into two equal halves and passes through the exact three dimensional center of the globe. This bracelet is a great circle on the globe and it represents a great circle on the real Earth.
Next imagine that you can slip and slide this bracelet anywhere you want on your globe provided it stays in full contact with the globe. In other words if you slide the near side up the back side slides down, but the whole bracelet stays in contact with the globe. The circumference of the globe and the circumference of the bracelet stay the same regardless of where you slide the bracelet because the globe is a sphere. No matter where you slip the bracelet on the globe it still has a circumference equal to the maximum circumference of the globe and so it always remains a great circle on the globe – and it always represents a great circle on Earth.
Realizing that the circumference of the bracelet never changes you mark a scale along it in degrees from 0° to 360° and now you can slide the bracelet anywhere you want on the globe to connect any two points and measure the distance between them in degrees.
Because the real Earth is (almost) a sphere and all of Earth's great circles have the same circumference we adopted the convention that on Earth one degree along a great circle is 60 nautical miles. That convention won't work on other planets because they don't have the same circumference as Earth, but for the moment we are only concerned with navigation on Earth. This convention was not arrived at by accident or coincidence – it was deliberately contrived for the purposes of navigation. If we can measure the angle between any two points on Earth along a great circle with our globe and bracelet we can now easily convert that measurement into nmi. What follows explains why we take the measure in degrees instead of just directly measuring it in nautical miles.
Now consider the celestial sphere way out beyond the surface of the Earth. The celestial sphere shares the exact same center as Earth's center. Therefore any flat plane that passes through Earth's center and defines a great circle on Earth can be extended out to the celestial sphere where it forms another great circle out there. In our globe and bracelet model this means that no matter where we slide our bracelet it not only represents a great circle on Earth, it also represents a matched great circle out on the celestial sphere. These two great circles work as a matched pair.
Consider a classic wagon wheel. Whatever the angle is between any two spokes on the rim it will be the same angle between those spokes at the hub – because they share the same center.
In our model if we take two places on Earth, A and B, and find their zenith points on the celestial sphere, then the angle between those two zenith points when measured along a great circle on the celestial sphere will be the same angle as points A and B are apart when measured along the matched great circle on Earth, provided we make the measurement in degrees of arc and not in linear measurements such as nautical miles.
It turns out this is very handy because in celestial navigation we need to figure out how far we are standing from a star's geographic position (GP). Usually the GP is very far away from us and below our horizon, so we can't see it to measure it directly – but we can see our zenith and we can see the star sitting directly over its own GP. The angle measure from the zenith over to the star along a celestial sphere great circle is exactly the same as the angle measure from beneath our feet over to the star's GP along an Earth great circle – and the Earth version is easily converted into nmi. If we could reliably take the measurement from our zenith over to the star we would know how far we were standing from the GP of the star and our immediate problem would be solved.
It turns out that it is difficult to measure using your precise zenith as a reference, but it is relatively easy to use the horizon, and with a few simple corrections determine the star's height above the true horizontal. (Ho) Next we apply the knowledge that the entire angle from the true horizontal to our zenith is always 90° and so the distance from the zenith to the star (called the zenith distance) must be whatever is left over from 90° after you subtract Ho. Zenith distance = (90° – Ho). And this is the angular distance from you to the star's GP which, when converted into nmi, allows you to plot a circle of position centered around the star's GP at a known radius in nautical miles.
Actually for most sight reduction methods we never state the zenith distance explicitly, nevertheless it is there inside the “mathematical black box” of whatever sight reduction method you choose to use. Ho is related to the observed zenith distance. Calculated altitude from an an assumed position (Hc) is related to the zenith distance from the assumed position to which we will to compare our Ho. When we combine them to find the intercept the 90° parts from each zenith distance term cancel each other out.
(90° – Hc) – (90° – Ho) => (90° – 90°) + (Ho – Hc) => (Ho – Hc)
The last thing we need to do is to convince ourselves that we are measuring the distance up from the horizon to the star along an actual great circle on the celestial sphere.
When we take a shot of a star we face in the exact direction as the light rays coming from the star. Since the star is directly over its own GP that is the exact same direction as from us to the GP. Next we go to some pains to hold the sextant vertical as we take our measurement.
There are two light rays coming to our eye through the sextant. One is from the star and the other is from the horizon. We rock the sextant to find the spot where the ray from the horizon is straight down beneath the ray from the star and that is when we take our measurement. One way to define “straight down” is to say directly towards the center of the Earth. So these two light rays form a flat plane, and that plane can be extended straight down to go through the center of the Earth – and extended all the way out to the celestial sphere. Because such a plane forms great circles on both spheres we conclude that we are indeed measuring Ho along a great circle on the celestial sphere aligned with the direction of the star, and that it corresponds exactly with the great circle from beneath our feet over to the GP of the star.
I suppose you don't need to know all of this to practice celestial, and some people may find it a bit too esoteric while others may find my math too simple. But it does explain how it actually works. It was a great help to me when I finally figured it out and perhaps it will be a help to others. I know none of the texts I have explain it like this, which I hope is straightforward enough for most people to understand if they ponder it for a bit.
Other aspects that are germane to great circles also helped me a great deal when I studied the sailings – and that all began with the simple globe-and-bracelet mental model I've outlined above. But that is a discussion for another day.
PeterB
|
|
|
About (Daylight) Time |
Posted by: PeterB - 04-03-2022, 05:08 PM - Forum: General Topics Here
- No Replies
|
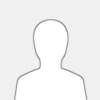 |
The US senate has recently passed an article to make Daylight Savings Time (summer time when the clocks are set one hour ahead) permanent. This isn't the first time the USA has experimented with this concept. They did it in January of 1974 to October of 1975. There was an oil crisis going on at that time and the idea was that this would somehow save heating oil because of the “longer” afternoon daylight. It all sounded like a good idea but once implemented it was disastrous. People hated it because they had to get up in the cold and dark on winter mornings to go to work or school. They found the dark mornings far more objectionable than changing their clocks. The action was soon repealed and we returned to springing forward and falling back.
I remember that experiment with our system of time and I recall it as being totally miserable in those winter months.
However, I suppose it is all a matter of what you are used to. I live near the eastern edge of the USA Eastern Time zone. My Wife's family comes from close to the western edge of the same time zone. When we go to visit them we are always acutely aware of the nearly 1 hour later sunrises (and sunsets) at the western edge of the same time zone.
In a related bit of trivia Paris, which is almost due south of Greenwich, runs one hour ahead of UTC (GMT) in the winter and then they set their clocks ahead another hour to European Summer Time in the summer months. In Paris when your watch says its almost noon in the summer the sun will be about 30° east of the meridian – a time most of us would consider late morning. Artists say the light is somehow special in Paris – and now you know why.
Daylight Savings Time Article
PeterB
|
|
|
Venus in Daylight My First Time |
Posted by: PeterB - 03-21-2022, 05:21 PM - Forum: General Topics Here
- Replies (2)
|
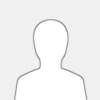 |
March 21 2022
Today is the unofficial first day of spring and it is the first day in many when my local skies have been clear.
Venus is visible during the daylight hours right now and will be for many more days. I had always heard about spotting Venus during the day but personally I never had much luck with it. A few days ago out of frustration I carefully calculated the correct azimuth and altitude from my back yard for a pre-selected observation time -- and then of course the clouds, rain and fog set in! Finally this morning it was beautifully clear. A few minor updates to my calculations and I had a pretty good idea where to look in the sky.
I saw nothing with my naked eye at first. I scanned the area systematically with a good set of 7 X 50 binoculars and Venus popped into view after a few passes, but I still couldn't find it with my naked eyes.
I wondered if I would be able to see it in a sextant scope so I fetched my Simex Mk 1 with a 4 -40 star scope and tried with that -- no luck
Eventually Venus moved enough in my local sky that I was able to fix its location against a tree branch in the foreground and then, with a little patience, and being careful to rest my head against a wall as I sat on my back porch so as to not move at all, I was able to pick up Venus with my naked eyes.
Once I had a naked eye view I could get it easily into the 4x40 scope but it was faint enough that it was still difficult to bring down. I didn't have a real horizon, only a fence rail, so this wasn't going to be an accurate sight, just fun practice.
I switched over to my humble Davis Mk3 "Lifeboat" sextant with a 0 power sight tube and therefore a huge field of view, and with that simple sextant it was possible to bring Venus down to my fence rail.
The trickiest part was to get to the point of picking it up with my naked eyes. This required a perfectly fixed position for myself and my head for the first cut. Once I had acquired it naked eye I easily re-acquired it naked eye -- at least for about 15 or 20 minutes.
All in all I was pleased to be able to engage in this exercise in my back yard, but I'm glad I wouldn't have to rely upon it from a moving, rocking boat! That would be very tough!
PeterB
|
|
|
Sight Calc App for Sight Reduction |
Posted by: PeterB - 03-18-2022, 08:10 PM - Forum: The Sight Reduction process
- No Replies
|
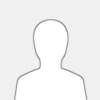 |
There is a free app available for smart phones called
"Sight Calc"
It is very straight forward and will provide you an azimuth, a true azimuth, and a calculated altitude for any input of Latitude, Longitude, LHA, and declination. It does not plot lines of position nor does it have any ephemeris data.
Since it works for all latitudes and longitudes you can input either a DR location or an Assumed Position. Using it with an AP makes it an excellent way to check your work when you are learning to use traditional sight reduction tables such as H.O. 229 or H.O. 249.
Its beauty is its simplicity -- and its price!
PeterB
|
|
|
Long by chron |
Posted by: neil_s - 02-16-2022, 08:29 PM - Forum: The Sight Reduction process
- Replies (1)
|
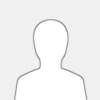 |
Rumata's post just below got me thinking about the Longitude by chronometer method, where you start with a DR Latitude. Your sun sight allows you to calculate the LHA. The difference between that and GHA from the almanac gives the Longitude. I am still bemused by the process of taking the Long by chron in the morning and then having to correct it when you take the meridian altitude at noon. Why not take it in the afternoon, when you already have the noon Latitude? I guess the master wanted a noon position and no other time would do! Anyway, you might have noticed the Endurance 22 expedition to find the wreck of Shackleton's ship in the Weddell sea. Three guys, Bergman, Mearns and Stuart have written a pertinent paper examining errors that may have been incorporated into the measurements that Frank Worsley, the ship's Master, made to fix the position when she sank. They site errors in the star maps and nautical almanac for 1915 and suggest that the ship will lie about 3 Km away from Worsley's position. To get back to Long by chron - this was the method Frank Worsley was using and in their paper, Bergman, Mearns and Stuart say "Ideally, the time sight should be made when the body being observed lies on the prime vertical, due East or West as the longitude obtained is independent of the estimated latitude and any uncertainties therein" Am I right, then in thinking that this method not only gives your position without having to do any plotting, but also is not sensitive to the latitude estimate, if you shoot the Sun when it lies due East? Bergman, Mearns and Stuart observe that Worsley did not do this, even though the Sun would have been high enough in the sky for a good sight. Long by chron might be making a comeback!
|
|
|
Sextant - what are these bits for? |
Posted by: neil_s - 01-30-2022, 08:32 PM - Forum: General Topics Here
- Replies (6)
|
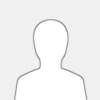 |
Hi to all - I am new on the site and a relatively new self taught celestial navigator - I've been hooked for about three years, now. There are a couple of things that are bugging me about using a sextant that the books don't seem to answer. I started out with an Ebbco plastic with bits of film negative where the filters should be and got on pretty fine with that. I coped with measuring ie for each observation, and found that multiple shots and averaging would give LOP's pretty close to where the GPS said. Now, I have a rather better instrument. I don't need to average any more, but am puzzled about the choice of filters. The direct sunlight ones I understand, but what are the green and blue ones for? Another thing is the choice of telescopes. I have only a 4X30 on my sextant but I see that a long thin X12 telescope was also offered. What was that used for? I also see that more modern instruments have telescopes with prisms, maybe 7X30. Do these have any advantage over my 4X30? All comments most welcome!
|
|
|
Article about CN vs GPS. |
Posted by: Rumata - 12-14-2021, 01:14 AM - Forum: General Topics Here
- No Replies
|
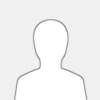 |
Gentlemen, I found this article of a general interest. Admittedly, a lot of stuff you know. But anyway, I found it more read-provoking than listening to MSNBC.
-----------------------------------------------------------------------------------------------------
Twenty-five years ago, in 1995, the U.S. Air Force reached a milestone: Its satellite Global Positioning System became fully operational. Today, GPS directs our world, from supertankers to pizza deliveries. But before the creation of this modern navigational marvel, humans looked to the heavens to find their way.
For centuries, using a sextant, a sailor could mark a ship’s location by making a few calculations to determine a star’s position relative to the horizon. Now a high-tech version of this maritime tradition is finding its way back into practice.
GPS is indispensable these days—but it’s still incredibly fragile. It can be spoofed with a fake satellite signal, hacked by an adversary, or simply destroyed. Spoofing, hacking, or destroying the stars? Not happening.
GPS may have revolutionized the way we navigate, but for years celestial navigation has been undergoing a quiet revolution of its own.
[font="Placard W01", Helvetica, Arial, sans-serif]DON’T CALL IT A COMEBACK[/font]
The U.S. Navy became less reliant on the traditional sextant back in WWII with the advent of radio navigation tools, but the heavens have remained an important map for sailors and especially pilots. WWII bombers like the B-17 Flying Fortress had an ‘astrodome,’ a transparent hemisphere that allowed the navigator to take star sightings.
![[Image: b-17-on-bomb-run-1618852590-ab-161909473...size=480:*]](https://hips.hearstapps.com/hmg-prod.s3.amazonaws.com/images/b-17-on-bomb-run-1618852590-ab-1619094734.jpg?resize=480:*)
[font="Freight Sans Pro W01", Helvetica, Arial, sans-serif]The astrodome, found on the nose of the B-17G in front of the cockpit window, helped navigators find their way using the stars.[/font]
[font="Freight Sans Pro W01", Helvetica, Arial, sans-serif]SMITHSONIAN INSTITUTIONWIKIMEDIA COMMONS[/font]
After the war and the birth of the rocket engine, scientists set about giving missiles the same kind of celestial smarts.
The SM-62 Snark cruise missile, developed in the 1950s, featured the first automated celestial navigation system, a mechanical-electrical device that weighed almost a ton. The Snark’s navigator had a small telescope mounted on three gimbals that rotated to point it to a given area of the sky. An early photosensor located the stars in the field of view and measured their angles, identifying them from a star catalog. The makers claimed that the Snark was able to navigate to within about three kilometers, but it never worked that well in practice.
Then again, you don’t need much navigation finesse when you’re delivering a 3.8-megaton nuclear warhead.
[font="Freight Sans Pro W00", Helvetica, Arial, sans-serif][b]How a Sextant Works[/b][/font]
![[Image: pop-astro-nav-illo-1619057122.jpg?resize=480:*]](https://hips.hearstapps.com/hmg-prod.s3.amazonaws.com/images/pop-astro-nav-illo-1619057122.jpg?resize=480:*)
[font="Freight Sans Pro W01", Helvetica, Arial, sans-serif]PRINT COLLECTIONGETTY IMAGES[/font]
Quote:It may look daunting, but a sextant simply measures the angle between a heavenly body—star, planet, moon, or sun—and the horizon.
Quote:Key components of a sextant are a miniature telescope, a half-silvered mirror (through which the telescope looks), and an index mirror on a moving arm. Looking at a celestial body through the telescope, you rotate the index mirror until it appears to be on the horizon. You then note the angle of the arm that corresponds to the angle of the celestial body from the horizon.
Quote:Measuring the angle from one heavenly body tells navigators that they are somewhere on a circle on the Earth’s surface, known as the position circle. For example, at any given moment there are a range of positions from which the sun is at exactly 35 degrees, lying on a circle about 3,000 miles in diameter. Navigators can plot this circle on the map if they have the relevant charts and know the exact time.
Quote:Because the circle is so large, it appears as a straight line on a local map. Plotting two such circles for two celestial objects gives two lines, and where they intersect is your location—in theory. Careful navigators usually take three or more sightings, because the lines do not necessarily meet at a single point. Three lines enclose a triangular area of possible positions traditionally known as a ‘cocked hat.'
Decades later, engineers built modernized celestial navigators, now about the size of a microwave oven, into the B-2 bomber and Trident missile. An observatory at Boeing’s Guidance Repair Center in Heath, Ohio, checks the location of the North Star every few days, and uses the measurements to calibrate military navigation and guidance systems. In modern missiles, celestial guidance is used in conjunction with an inertial navigation system (INS).
While celestial navigation survived in niche applications like missiles, GPS has edged out the competition because it’s accurate [i]and[/i] cheap[i]. [/i]But GPS works only when there’s a good satellite signal, and adversaries are very much aware of that weakness.
[font="Placard W01", Helvetica, Arial, sans-serif]GPS: REVOLUTIONARY…WHEN IT WORKS[/font]
In 2018, Russian GPS jamming disrupted a NATO exercise in Scandinavia, and Chinese GPS spoofing signals can cause ships in many coastal areas to see false locations. Modern military GPS systems feature a range of electronic guards to prevent jamming and spoofing, including directional antennas and a special M-code military satellite signal.
Even with these safeguards, a GPS signal may simply not be there: There are concerns about whether the satellite system might be hacked, or the satellites themselves knocked out by an enemy or a massive solar storm. This has focused military minds on alternatives.
![[Image: gettyimages-1061224044.jpeg?crop=0.66676...size=480:*]](https://hips.hearstapps.com/hmg-prod.s3.amazonaws.com/images/gettyimages-1061224044.jpeg?crop=0.6667600896860987xw:1xh;center,top&resize=480:*)
[font="Freight Sans Pro W01", Helvetica, Arial, sans-serif]A soldier checks a map during NATO’s Trident Juncture exercise in October 2018. Participants accused the Russian government of GPS jamming during the exercise.[/font]
[font="Freight Sans Pro W01", Helvetica, Arial, sans-serif]LEON NEALGETTY IMAGES[/font]
The Navy’s Automated Celestial Navigation System would replace manual shipboard measurements with something more accurate, while Special Operations Command experts are developing a handheld device for commandos. Both pieces of tech are aiming for GPS-level precision.
“The best accuracy for celestial navigation with certainty is within a couple of meters,” says Benjamin Lane of the Advanced Position, Navigation & Timing Instrumentation unit at Draper Laboratory in Cambridge, Massachusetts. “In practice, we are within a factor of a few of that.”
Quote:[font="Placard W01", Helvetica, Arial, sans-serif]SPOOFING, HACKING, OR DESTROYING THE STARS? NOT HAPPENING.[/font]
The new systems use infrared rather than visible light for locating stars, allowing daytime navigation. The stars shine just as brightly in the day sky as they do at night, but their light is masked by sunlight scattered by the atmosphere. The scattering is strongest at short wavelengths. If you’ve ever glimpsed the sky, you know that blue light is scattered the most. But glimpse that same sky with a filter that allows only infrared light, and the sky suddenly becomes dark—and filled with stars.
“Twenty years ago, these infrared sensors were quite expensive,” says George Kaplan, a consultant for the U.S. Naval Observatory. “Since then, costs have been going down and the pixel count is getting higher.”
The rise of celestial navigation is also helped along with another piece of tech called a “phased optical array,” which does not need to be pointed at a section of the sky like a telescope does. This type of sensor does not have a lens to focus, but instead has an array of tiny meta-material antennas to capture light. A processing unit can phase-shift the signal from each antenna to achieve the same effect as a movable, focusing lens.
Lane and his team have already demonstrated a working prototype of such a sensor.
![[Image: gettyimages-1211085530.jpeg?crop=1.00xw:...size=480:*]](https://hips.hearstapps.com/hmg-prod.s3.amazonaws.com/images/gettyimages-1211085530.jpeg?crop=1.00xw:0.923xh;0,0.0204xh&resize=480:*)
[font="Freight Sans Pro W01", Helvetica, Arial, sans-serif]A view of SpaceX’s Starlink constellation at night.[/font]
[font="Freight Sans Pro W01", Helvetica, Arial, sans-serif]YURI SMITYUKGETTY IMAGES[/font]
[font="Placard W01", Helvetica, Arial, sans-serif]TWINKLE, TWINKLE, LITTLE SATELLITE[/font]
But the biggest development, being pursued by both Draper Laboratories and Trex Enterprises, is an approach that overcomes one of the biggest limits to accuracy. Traditional celestial navigation requires measuring the angle of a star or planet in the sky. To do this you need to precisely locate the horizon or, alternatively, the azimuth or true vertical.
That sounds simple, but it isn't.
“Trex has tried infrared detection of the horizon,” says Kaplan. “The problem is that the horizon is refractive; it’s very sensitive to temperature differences. You get all sorts of odd reflections, including mirage.”
Others have sensed the azimuth by the polarization of the sky, or by the refraction of starlight, or even angular motion sensors detecting the Earth’s rotation. None of them comes close to GPS accuracy. But this new approach takes readings from human-made satellites rather than stars, sidestepping the horizon altogether.
[font="Freight Sans Pro W01", Helvetica, Arial, sans-serif]A Military History of Celestial Navigation[/font]
[font="Freight Sans Pro W00", Helvetica, Arial, sans-serif]Longines Weems A-11 Aviators Watch
The precise time is vital for celestial navigation: an uncertainty of 30 seconds means a distance error of ten kilometers. The Dutch aviator Capt. PVH Weems developed a watch with a second hand that could be set exactly to radio time signal broadcasts, which the U.S. Navy started in 1904, reducing errors. The U.S. Army Air Corps commissioned its own version of the watch in 1937, the A-11, and issued one to every new aviator.
[font="Freight Sans Pro W00", Helvetica, Arial, sans-serif]Plexiglas Astrodome[/font]
Introduced just in time for WWII bombers, the astrodome was a wonder of materials technology: Plexiglas, a rugged transparent plastic, formed into a dome giving a clear 360-degree view of the night sky. The astrodome included a hanging point for a sextant so navigators would not have to hold the weighty instrument.
[font="Freight Sans Pro W00", Helvetica, Arial, sans-serif]Fairchild-Maxson Mark I Line of Position Computer[/font]
Calculating position from star readings was a time-consuming and demanding task—there was no ‘app for that’ in pre-electronic days. The USAAF automated the process with this 1938 mechanical calculating device. The navigator dialed in the angle readings and the time, and the 20-pound computer did the rest. Several dozen were built and used by the Air Corps and the Navy, but the design proved too expensive.
[font="Freight Sans Pro W00", Helvetica, Arial, sans-serif]U-2 Driftsight[/font]
The dashboard of the U-2 spy plane was dominated by the driftsight, a downward-looking periscope to follow roads and other landmarks for navigation. When the ground was invisible, flipping a mirror converted the driftsight into a sextant with a view of the sky through a glass bubble on the plane’s nose. This was helpful in areas like the Arctic with no terrain features and where magnetic compasses were useless.
[font="Freight Sans Pro W00", Helvetica, Arial, sans-serif]Nortronics NAS-14V2 Navigation System[/font]
Affectionately known as R2-D2, the celestial navigator in the SR-71 Blackbird occupied a position immediately behind the pilot. Measuring 48 x 46 x 31 in. and weighing 300 pounds, it performed the vital function of keeping the Blackbird on track even at Mach 3, ensuring that the plane’s cameras photographed the right area of interest in the ground. It also helped keep the valuable plane on the safe side of sensitive international borders.[/font]
Because satellites are relatively close to the Earth’s surface, observers can calculate their own position based on the angles among different satellites. The navigator does not need to see the horizon or know the local vertical. Instead, it’s a version of triangulation that any hiker uses when taking angle readings from two mountaintops.
Any visible satellite can be used to navigate if its position is known, and there are now plenty to choose from. In 2010, fewer than 1,000 satellites were in orbit; that number has more than doubled and is rising fast with mass launches of small satellites. SpaceX’s Starlink constellation alone will add more than 7,000 in the next few years, so there should always be one handy to take a reading.
“Starlink’s satellites are being made less reflective but still trackable,” says Lane. “If they are predictable, then we can track and use them.”
The only limit is how accurately a satellite’s position is known. While the orbital track is usually known exactly, a satellite’s position along the orbital track is affected by atmospheric drag, resulting in a deviation of arc minutes (an error of one nautical mile, or 1,952 meters.)
Fortunately, the North American Aerospace Defense Command (NORAD) tracks many of them with high-precision radar, and can supply the data to military users.
Once these celestial navigation tools are ready for combat, they’ll come in different shapes and sizes. The B-21 Raider bomber will likely have a more compact, highly precise version of the B-2 Spirit’s R2-D2-like unit that sits behind the pilot. On Navy ships, the Automated Celestial Navigation System will replace the old brass sextants and provide data that can be fed into shipboard computers along with other navigational sensors. Future ballistic missiles will also include advanced celestial navigation.
And with a new handheld device being developed by Special Operations Command, even the humble foot soldier could be steering by the stars.
[font="Placard W01", Helvetica, Arial, sans-serif]CELESTIAL LIMITATIONS[/font]
![[Image: 348058-1618867837.jpeg?crop=0.726xw:1.00...size=480:*]](https://hips.hearstapps.com/hmg-prod.s3.amazonaws.com/images/348058-1618867837.jpeg?crop=0.726xw:1.00xh;0.136xw,0&resize=480:*)
[font="Freight Sans Pro W01", Helvetica, Arial, sans-serif]Senior Chief Petty Officer Jonathan Myers teaches Command Master Chief April Beldo how to use a marine sextant on the USS [i]Carl Vinson[/i].[/font]
[font="Freight Sans Pro W01", Helvetica, Arial, sans-serif]TRAVIS K. MENDOZA[/font]
While it is already possible to get a location within 20 meters or so, and devices with more accuracy are in the pipeline, celestial navigation is not quite as handy as GPS. For one thing, it currently takes much longer to get a reading, often tens of seconds.
For another, Kaplan says that even though infrared imagers work through haze and light cloud cover, they cannot see through dense, low clouds. Unlike GPS, celestial navigation will not be available 24/7, so it would have to work with an inertial navigation system. Luckily, these are getting much smaller and cheaper too.
“Another ‘gotcha’ using LEO satellites is that in daytime the light is coming from above,” says Kaplan. This means the brightest part, the solar array, is pointed away from the Earth and the satellite is much less bright than it is at night. But the developers are confident that they can overcome these issues, unlocking celestial navigation’s true potential as a GPS substitute—without all the dedicated space hardware, risks of jamming, or vulnerability to cyberattack.
In 1980, the first portable Rockwell GPS receiver was a backpack unit that weighed 20 pounds. It took five minutes to get a fix and cost around $30,000. Modern GPS receivers are chip-sized, give an almost instant readout, and cost less than $50.
In 40 years, who knows where the stars might take us.
|
|
|
Sign of the times. |
Posted by: pabrides - 06-24-2021, 12:42 PM - Forum: General Topics Here
- Replies (1)
|
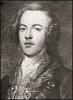 |
I just thought I'd check in to see if anyone has been using their sextants lately.
It's rainy season in pi so the number of observation days is limited, but I vow to get something this week even if I have to guess at it.
Any other news out there?
Can't wait to hear from my CN friends...
Cheers
|
|
|
|